The terms dominant and recessive describe the inheritance patterns of certain traits. That is, they describe how likely it is for a certain phenotype to pass from parent offspring.
Sexually reproducing species, including people and other animals, have two copies of each gene. The two copies, called alleles, can be slightly different from each other. The differences can cause variations in the protein that’s produced, or they can change protein expression: when, where, and how much protein is made. Proteins affect traits, so variations in protein activity or expression can produce different phenotypes.
A dominant allele produces a dominant phenotype in individuals who have one copy of the allele, which can come from just one parent. For a recessive allele to produce a recessive phenotype, the individual must have two copies, one from each parent. An individual with one dominant and one recessive allele for a gene will have the dominant phenotype. They are generally considered “carriers” of the recessive allele: the recessive allele is there, but the recessive phenotype is not.
The terms are confusing and often misleading
Dominant and recessive inheritance are useful concepts when it comes to predicting the probability of an individual inheriting certain phenotypes, especially genetic disorders. But the terms can be confusing when it comes to understanding how a gene specifies a trait. This confusion comes about in part because people observed dominant and recessive inheritance patterns before anyone knew anything about DNA and genes, or how genes code for proteins that specify traits.
The critical point to understand is that there is no universal mechanism by which dominant and recessive alleles act. Dominant alleles do not physically “dominate” or “repress” recessive alleles. Whether an allele is dominant or recessive depends on the particulars of the proteins they code for.
The terms can also be subjective, which adds to the confusion. The same allele can be considered dominant or recessive, depending on how you look at it. The sickle-cell allele, described below, is a great example.
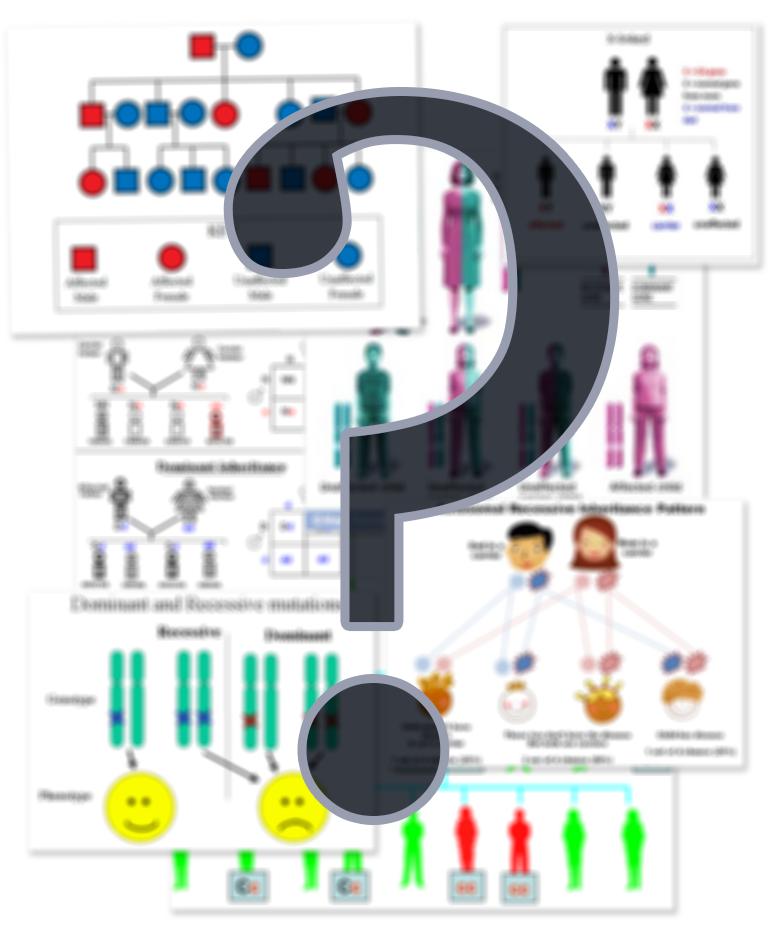
Do a simple internet search, and you’ll find pages and pages of charts, images, and text explaining dominant and recessive inheritance patterns. However, these patterns apply to few traits.
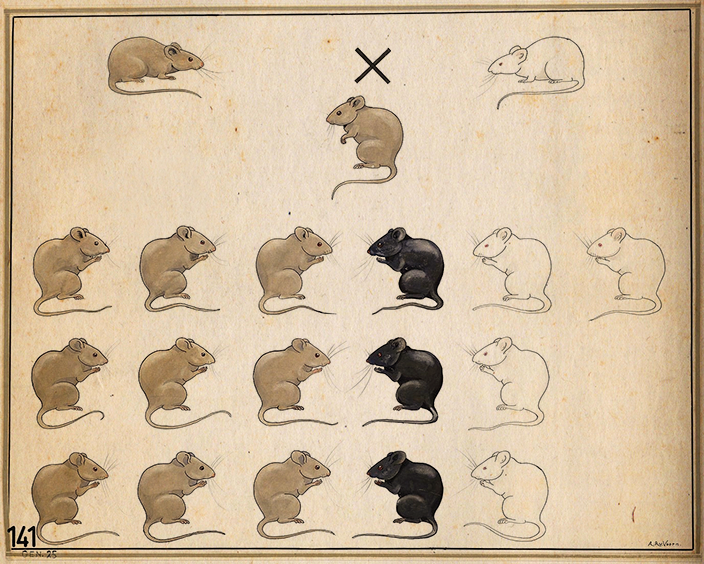